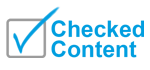
Bohrium
About this schools Wikipedia selection
This wikipedia selection has been chosen by volunteers helping SOS Children from Wikipedia for this Wikipedia Selection for schools. Child sponsorship helps children one by one http://www.sponsor-a-child.org.uk/.
Bohrium | |||||||||||||||||||||||||||||||||||||||||||
---|---|---|---|---|---|---|---|---|---|---|---|---|---|---|---|---|---|---|---|---|---|---|---|---|---|---|---|---|---|---|---|---|---|---|---|---|---|---|---|---|---|---|---|
107Bh
|
|||||||||||||||||||||||||||||||||||||||||||
|
|||||||||||||||||||||||||||||||||||||||||||
Appearance | |||||||||||||||||||||||||||||||||||||||||||
unknown | |||||||||||||||||||||||||||||||||||||||||||
General properties | |||||||||||||||||||||||||||||||||||||||||||
Name, symbol, number | bohrium, Bh, 107 | ||||||||||||||||||||||||||||||||||||||||||
Pronunciation | / ˈ b ɔər i ə m / | ||||||||||||||||||||||||||||||||||||||||||
Metallic category | transition metal | ||||||||||||||||||||||||||||||||||||||||||
Group, period, block | 7, 7, d | ||||||||||||||||||||||||||||||||||||||||||
Standard atomic weight | [270] | ||||||||||||||||||||||||||||||||||||||||||
Electron configuration | [Rn] 5f14 6d5 7s2 (calculated) 2, 8, 18, 32, 32, 13, 2 (predicted) |
||||||||||||||||||||||||||||||||||||||||||
History | |||||||||||||||||||||||||||||||||||||||||||
Discovery | Gesellschaft für Schwerionenforschung (1981) | ||||||||||||||||||||||||||||||||||||||||||
Physical properties | |||||||||||||||||||||||||||||||||||||||||||
Phase | solid (predicted) | ||||||||||||||||||||||||||||||||||||||||||
Density (near r.t.) | 37 (predicted) g·cm−3 | ||||||||||||||||||||||||||||||||||||||||||
Atomic properties | |||||||||||||||||||||||||||||||||||||||||||
Oxidation states | 7, 5, 4, 3 (predicted) (only bolded oxidation states are known experimentally) |
||||||||||||||||||||||||||||||||||||||||||
Ionization energies ( more) |
1st: 742.9 (estimated) kJ·mol−1 | ||||||||||||||||||||||||||||||||||||||||||
2nd: 1688.5 (estimated) kJ·mol−1 | |||||||||||||||||||||||||||||||||||||||||||
3rd: 2566.5 (estimated) kJ·mol−1 | |||||||||||||||||||||||||||||||||||||||||||
Atomic radius | 128 (predicted) pm | ||||||||||||||||||||||||||||||||||||||||||
Covalent radius | 141 (estimated) pm | ||||||||||||||||||||||||||||||||||||||||||
Miscellanea | |||||||||||||||||||||||||||||||||||||||||||
CAS registry number | 54037-14-8 | ||||||||||||||||||||||||||||||||||||||||||
Most stable isotopes | |||||||||||||||||||||||||||||||||||||||||||
Main article: Isotopes of bohrium | |||||||||||||||||||||||||||||||||||||||||||
|
|||||||||||||||||||||||||||||||||||||||||||
Bohrium is a chemical element with symbol Bh and atomic number 107, named in honour of Danish physicist Niels Bohr. It is a synthetic element (an element that can be created in a laboratory but is not found in nature) and radioactive; the most stable known isotope, 270Bh, has a half-life of approximately 61 seconds.
In the periodic table of the elements, it is a d-block transactinide element. It is a member of the 7th period and belongs to the group 7 elements. Chemistry experiments have confirmed that bohrium behaves as the heavier homologue to rhenium in group 7. The chemical properties of bohrium are characterized only partly, but they compare well with the chemistry of the other group 7 elements.
History

Official discovery
Bohrium was first convincingly synthesized in 1981 by a German research team led by Peter Armbruster and Gottfried Münzenberg at the Institute for Heavy Ion Research (Gesellschaft für Schwerionenforschung) in Darmstadt. The team bombarded a target of bismuth-209 with accelerated nuclei of chromium-54 to produce 5 atoms of the isotope bohrium-262:
- 209
83Bi + 54
24Cr → 262
107Bh + n
The IUPAC/IUPAP Transfermium Working Group (TWG) recognised the GSI collaboration as official discoverers in their 1992 report.
Proposed names
The German group suggested the name nielsbohrium with symbol Ns to honour the Danish physicist Niels Bohr. The Soviet scientists at the Joint Institute for Nuclear Research in Dubna, Russia had suggested this name be given to element 105 (which was finally called dubnium) and the German team wished to recognise both Bohr and the fact that the Dubna team had been the first to propose the cold fusion reaction to solve the controversial problem of the naming of element 105. The Dubna team agreed with the German group's naming proposal for element 107.
There was an element naming controversy as to what the elements from 104 to 106 were to be called; the IUPAC adopted unnilseptium (symbol Uns) as a temporary, systematic element name for this element. In 1994 a committee of IUPAC recommended that element 107 be named bohrium, not nielsbohrium, since there was no precedence for using a scientist's complete name in the naming of an element. This was opposed by the discoverers as there was some concern that the name might be confused with boron and in particular the distinguishing of the names of their respective oxoanions, bohrate and borate. The matter was handed to the Danish branch of IUPAC which, despite this, voted in favour of the name bohrium, and thus the name bohrium for element 107 was recognized internationally in 1997. The IUPAC subsequently decided that bohrium salts should be called bohriates instead of bohrates.
Nucleosynthesis
Super-heavy elements such as bohrium are produced by bombarding lighter elements in particle accelerators that induce fusion reactions. Whereas most of the isotopes of bohrium can be synthesized directly this way, some heavier ones have only been observed as decay products of elements with higher atomic numbers.
Depending on the energies involved, the former are separated into "hot" and "cold". In hot fusion reactions, very light, high-energy projectiles are accelerated toward very heavy targets (actinides), giving rise to compound nuclei at high excitation energy (~40–50 MeV) that may either fission or evaporate several (3 to 5) neutrons. In cold fusion reactions, the produced fused nuclei have a relatively low excitation energy (~10–20 MeV), which decreases the probability that these products will undergo fission reactions. As the fused nuclei cool to the ground state, they require emission of only one or two neutrons, and thus, allows for the generation of more neutron-rich products. The latter is a distinct concept from that of where nuclear fusion claimed to be achieved at room temperature conditions (see cold fusion).
Cold fusion
Before the first successful synthesis of hassium in 1981 by the GSI team, the synthesis of bohrium was first attempted in 1976 by scientists at the Joint Institute for Nuclear Research at Dubna using this cold fusion reaction. They detected two spontaneous fission activities, one with a half-life of 1-2 ms and one with a half-life of 5 s. Based on the results of other cold fusion reactions, they concluded that they were due to 261Bh and 257Db respectively. However, later evidence gave a much lower SF branching for 261Bh reducing confidence in this assignment. The assignment of the dubnium activity was later changed to 258Db, presuming that the decay of bohrium was missed. The 2 ms SF activity was assigned to 258Rf resulting from the 33% EC branch. The GSI team studied the reaction in 1981 in their discovery experiments. Five atoms of 262Bh were detected using the method of correlation of genetic parent-daughter decays. In 1987, an internal report from Dubna indicated that the team had been able to detect the spontaneous fission of 261Bh directly. The GSI team further studied the reaction in 1989 and discovered the new isotope 261Bh during the measurement of the 1n and 2n excitation functions but were unable to detect an SF branching for 261Bh. They continued their study in 2003 using newly developed bismuth(III) fluoride (BiF3) targets, used to provide further data on the decay data for 262Bh and the daughter 258Db. The 1n excitation function was remeasured in 2005 by the team at the Lawrence Berkeley National Laboratory (LBNL) after some doubt about the accuracy of previous data. They observed 18 atoms of 262Bh and 3 atoms of 261Bh and confirmed the two isomers of 262Bh.
In 2007, the team at LBNL studied the analogous reaction with chromium-52 projectiles for the first time to search for the lightest bohrium isotope 260Bh:
- 209
83Bi + 52
24Cr → 260
107Bh + n
The team successfully detected 8 atoms of 260Bh decaying by alpha decay to 256Db, emitting alpha particles with energy 10.16 MeV. The alpha decay energy indicates the continued stabilizing effect of the N=152 closed shell.
The team at Dubna also studied the reaction between lead-208 targets and manganese-55 projectiles in 1976 as part of their newly established cold fusion approach to new elements:
- 208
82Pb + 55
25Mn → 262
107Bh + n
They observed the same spontaneous fission activities as those observed in the reaction between bismuth-209 and chromium-54 and again assigned them to 261Bh and 257Db. Later evidence indicated that these should be reassigned to 258Db and 258Rf (see above). In 1983, they repeated the experiment using a new technique: measurement of alpha decay from a decay product that had been separated out chemically. The team were able to detect the alpha decay from a decay product of 262Bh, providing some evidence for the formation of bohrium nuclei. This reaction was later studied in detail using modern techniques by the team at LBNL. In 2005 they measured 33 decays of 262Bh and 2 atoms of 261Bh, providing an excitation function for the reaction emitting one neutron and some spectroscopic data of both 262Bh isomers. The excitation function for the reaction emitting two neutrons was further studied in a 2006 repeat of the reaction. The team found that the reaction emitting one neutron had a higher cross section than the corresponding reaction with a 209Bi target, contrary to expectations. Further research is required to understand the reasons.
Hot fusion
The reaction between uranium-238 targets and phosphorus-31 projectiles was first studied in 2006 at the LBNL as part of their systematic study of fusion reactions using uranium-238 targets:
- 238
92U + 31
15P → 264
107Bh + 5 n
Results have not been published but preliminary results appear to indicate the observation of spontaneous fission, possibly from 264Bh.
Recently, the team at the Institute of Modern Physics (IMP), Lanzhou, have studied the nuclear reaction between americium-243 targets and accelerated nuclei of magnesium-26 in order to synthesise the new isotope 265Bh and gather more data on 266Bh:
- 243
95Am + 26
12Mg → 269−x
107Bh + x n (x = 3, 4, or 5)
In two series of experiments, the team measured partial excitation functions for the reactions emitting three, four, and five neutrons.
The reaction between targets of curium-248 and accelerated nuclei of sodium-23 was studied for the first time in 2008 by the team at RIKEN, Japan, in order to study the decay properties of 266Bh, which is a decay product in their claimed decay chains of ununtrium:
- 248
96Cm + 23
11Na → 271−x
107Bh + x n (x = 4 or 5)
The decay of 266Bh by the emission of alpha particles with energies of 9.05-9.23 MeV was further confirmed in 2010.
The first attempts to synthesize bohrium by hot fusion pathways were performed in 1979 by the team at Dubna, using the reaction between accelerated nuclei of neon-22 and targets of berkelium-249:
- 249
97Bk + 22
10Ne → 271−x
107Bh + x n (x = 4 or 5)
The reaction was repeated in 1983. In both cases, they were unable to detect any spontaneous fission from nuclei of bohrium. More recently, hot fusions pathways to bohrium have been re-investigated in order to allow for the synthesis of more long-lived, neutron rich isotopes to allow a first chemical study of bohrium. In 1999, the team at LBNL claimed the discovery of long-lived 267Bh (5 atoms) and 266Bh (1 atom). Later, both of these were confirmed. The team at the Paul Scherrer Institute (PSI) in Bern, Switzerland later synthesized 6 atoms of 267Bh in the first definitive study of the chemistry of bohrium.
As decay products
Evaporation residue | Observed bohrium isotope |
---|---|
294Uus, 290Uup, 286Uut, 282Rg, 278Mt | 274Bh |
288Uup, 284Uut, 280Rg, 276Mt | 272Bh |
287Uup, 283Uut, 279Rg, 275Mt | 271Bh |
282Uut, 278Rg, 274Mt | 270Bh |
278Uut, 274Rg, 270Mt | 266Bh |
272Rg, 268Mt | 264Bh |
266Mt | 262Bh |
Bohrium has been detected in the decay chains of elements with a higher atomic number, such as meitnerium. Meitnerium currently has seven known isotopes; all of them undergo alpha decays to become bohrium nuclei, with mass numbers between 262 and 274. Parent meitnerium nuclei can be themselves decay products of roentgenium, ununtrium, ununpentium, or ununseptium. To date, no other elements have been known to decay to bohrium. For example, in January 2010, the Dubna team ( JINR) identified bohrium-274 as a product in the decay of ununseptium via an alpha decay sequence:
- 294
117Uus → 290
115Uup + 4
2He - 290
115Uup → 286
113Uut + 4
2He - 286
113Uut → 282
111Rg + 4
2He - 282
111Rg → 278
109Mt + 4
2He - 278
109Mt → 274
107Bh + 4
2He
Isotopes
Isotope |
Half-life |
Decay mode |
Discovery year |
Reaction |
---|---|---|---|---|
260Bh | 35 ms | α | 2007 | 209Bi(52Cr,n) |
261Bh | 11.8 ms | α | 1986 | 209Bi(54Cr,2n) |
262Bh | 84 ms | α | 1981 | 209Bi(54Cr,n) |
262mBh | 9.6 ms | α | 1981 | 209Bi(54Cr,n) |
263Bh | 0.2? ms | α ? | unknown | — |
264Bh | 0.97 s | α | 1994 | 272Rg(—,2α) |
265Bh | 0.9 s | α | 2004 | 243Am(26Mg,4n) |
266Bh | 0.9 s | α | 2000 | 249Bk(22Ne,5n) |
267Bh | 17 s | α | 2000 | 249Bk(22Ne,4n) |
268Bh | 25? s | α, SF? | unknown | — |
269Bh | 25? s | α ? | unknown | — |
270Bh | 61 s | α | 2006 | 282Uut(—,3α) |
271Bh | 1.2 s | α | 2003 | 287Uup(—,4α) |
272Bh | 9.8 s | α | 2005 | 288Uup(—,4α) |
273Bh | 90? min | α, SF ? | unknown | — |
274Bh | ~54 s | α | 2009 | 294Uus(—,5α) |
275Bh | 40? min | SF ? | unknown | — |
Bohrium has no stable or naturally-occurring isotopes. Several radioactive isotopes have been synthesized in the laboratory, either by fusing two atoms or by observing the decay of heavier elements. Eleven different isotopes of bohrium have been reported with atomic masses 260–262, 264–267, 270–272, 274, one of which, bohrium-262, has a known metastable state. All of these decay only through alpha decay, although some unknown bohrium isotopes are predicted to undergo spontaneous fission.
Stability and half-lives
The lighter isotopes usually have shorter half-lives; half-lives of under 100 ms for 260Bh, 261Bh, 262Bh, 262mBh, and 263Bh were observed. 264Bh, 265Bh, 266Bh, and 271Bh are more stable at around 1 s, and 267Bh and 272Bh have half-lives of about 10 s. The heaviest isotopes are the most stable, with 270Bh and 274Bh having measured half-lives of about 61 s and 54 s respectively. The unknown isotopes 273Bh and 275Bh are predicted to have even longer half-lives of around 90 minutes and 40 minutes respectively. Before its discovery, 274Bh was also predicted to have a long half-life of 90 minutes, but it was found to have a shorter half-life of only about 54 seconds.
The proton-rich isotopes with masses 260, 261, and 262 were directly produced by cold fusion, those with mass 262 and 264 were reported in the decay chains of meitnerium and roentgenium, while the neutron-rich isotopes with masses 266, 267 were created in irradiations of actinide targets. The four most neutron-rich ones with masses 270, 271, 272, and 274 appear in the decay chains of 282113, 287115, 288115, and 294117 respectively. These eleven isotopes have half-lives ranging from 8 miliseconds to 1 minute.
Nuclear isomerism
- 262Bh
The only confirmed example of isomerism in bohrium is in the isotope 262Bh. Direct synthesis of 262Bh results in two states, a ground state and an isomeric state. The ground state is confirmed to decay by alpha decay, emitting alpha particles with energies of 10.08, 9.82, and 9.76 MeV, and has a revised half-life of 84 ms. The excited state also decays by alpha decay, emitting alpha particles with energies of 10.37 and 10.24 MeV, and has a revised half-life of 9.6 ms.
Chemical properties
Extrapolated
Bohrium is projected to be the fourth member of the 6d series of transition metals and the heaviest member of group VII in the Periodic Table, below manganese, technetium and rhenium. All the members of the group readily portray their group oxidation state of +7 and the state becomes more stable as the group is descended. Thus bohrium is expected to form a stable +7 state. Technetium also shows a stable +4 state whilst rhenium exhibits stable +4 and +3 states. Bohrium may therefore show these lower states as well.
The heavier members of the group are known to form volatile heptoxides M2O7, so bohrium should also form the volatile oxide Bh2O7. The oxide should dissolve in water to form perbohric acid, HBhO4. Rhenium and technetium form a range of oxyhalides from the halogenation of the oxide. The chlorination of the oxide forms the oxychlorides MO3Cl, so BhO3Cl should be formed in this reaction. Fluorination results in MO3F and MO2F3 for the heavier elements in addition to the rhenium compounds ReOF5 and ReF7. Therefore, oxyfluoride formation for bohrium may help to indicate eka-rhenium properties.
Experimental
In 1995, the first report on attempted isolation of the element was unsuccessful.
In 2000, it was confirmed that although relativistic effects are important, the 107th element does behave like a typical group 7 element.
In 2000, a team at the PSI conducted a chemistry reaction using atoms of 267Bh produced in the reaction between Bk-249 and Ne-22 ions. The resulting atoms were thermalised and reacted with a HCl/O2 mixture to form a volatile oxychloride. The reaction also produced isotopes of its lighter homologues, technetium (as 108Tc) and rhenium (as 169Re). The isothermal adsorption curves were measured and gave strong evidence for the formation of a volatile oxychloride with properties similar to that of rhenium oxychloride. This placed bohrium as a typical member of group 7.
- 2 Bh + 3 O2 + 2 HCl → 2 BhO3Cl + H2
Formula | Name(s) |
---|---|
BhO3Cl | bohrium oxychloride ; bohrium(VII) chloride trioxide |