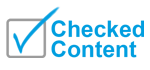
Chernobyl disaster
Did you know...
SOS Children have produced a selection of wikipedia articles for schools since 2005. Before you decide about sponsoring a child, why not learn about different sponsorship charities first?
![]() The nuclear reactor after the disaster. Reactor 4 (center). Turbine building (lower left). Reactor 3 (centre right). |
|
Date | 26 April 1986 |
---|---|
Time | 01:23 ( Moscow Time UTC+3) |
Location | ![]() |
The Chernobyl disaster ( Ukrainian: Чорнобильська катастрофа, Chornobylska Katastrofa – Chornobyl Catastrophe) was a catastrophic nuclear accident that occurred on 26 April 1986 at the Chernobyl Nuclear Power Plant in Ukraine (then officially Ukrainian SSR), which was under the direct jurisdiction of the central authorities of the Soviet Union. An explosion and fire released large quantities of radioactive particles into the atmosphere, which spread over much of Western USSR and Europe.
The Chernobyl disaster is widely considered to have been the worst nuclear power plant accident in history, and is one of only two classified as a level 7 event on the International Nuclear Event Scale (the other being the Fukushima Daiichi nuclear disaster in 2011). The battle to contain the contamination and avert a greater catastrophe ultimately involved over 500,000 workers and cost an estimated 18 billion rubles. The official Soviet casualty count of 31 deaths has been disputed, and long-term effects such as cancers and deformities are still being accounted for.
Summary
The disaster began during a systems test on Saturday, 26 April 1986 at reactor number four of the Chernobyl plant, which is near the city of Pripyat and in proximity to the administrative border with Belarus and the Dnieper river. There was a sudden and unexpected power surge, and when an emergency shutdown was attempted, an exponentially larger spike in power output occurred, which led to a reactor vessel rupture and a series of steam explosions. These events exposed the graphite moderator of the reactor to air, causing it to ignite. The resulting fire sent a plume of highly radioactive fallout into the atmosphere and over an extensive geographical area, including Pripyat. The plume drifted over large parts of the western Soviet Union and Europe. From 1986 to 2000, 350,400 people were evacuated and resettled from the most severely contaminated areas of Belarus, Russia, and Ukraine. According to official post-Soviet data, about 60% of the fallout landed in Belarus.
The accident raised concerns about the safety of the Soviet nuclear power industry, as well as nuclear power in general, slowing its expansion for a number of years and forcing the Soviet government to become less secretive about its procedures. The government coverup of the Chernobyl disaster was a "catalyst" for glasnost, which "paved the way for reforms leading to the Soviet collapse".
Russia, Ukraine, and Belarus have been burdened with the continuing and substantial decontamination and health care costs of the Chernobyl accident. A report by the International Atomic Energy Agency examines the environmental consequences of the accident. Another UN agency, UNSCEAR, has estimated a global collective dose of radiation exposure from the accident "equivalent on average to 21 additional days of world exposure to natural background radiation"; individual doses were far higher than the global mean among those most exposed, including 530,000 local recovery workers who averaged an effective dose equivalent to an extra 50 years of typical natural background radiation exposure each. Estimates of the number of deaths that will eventually result from the accident vary enormously; disparities reflect both the lack of solid scientific data and the different methodologies used to quantify mortality – whether the discussion is confined to specific geographical areas or extends worldwide, and whether the deaths are immediate, short term, or long term.
Thirty one deaths are directly attributed to the accident, all among the reactor staff and emergency workers. An UNSCEAR report places the total confirmed deaths from radiation at 64 as of 2008. The Chernobyl Forum estimates that the eventual death toll could reach 4,000 among those exposed to the highest levels of radiation (200,000 emergency workers, 116,000 evacuees and 270,000 residents of the most contaminated areas); this figure includes some 50 emergency workers who died of acute radiation syndrome, nine children who died of thyroid cancer and an estimated total of 3940 deaths from radiation-induced cancer and leukemia.
The Union of Concerned Scientists estimates that, among the hundreds of millions of people living in broader geographical areas, there will be 50,000 excess cancer cases resulting in 25,000 excess cancer deaths. For this broader group, the 2006 TORCH report predicts 30,000 to 60,000 excess cancer deaths, and a Greenpeace report puts the figure at 200,000 or more. The Russian publication Chernobyl concludes that among the billions of people worldwide who were exposed to radioactive contamination from the disaster, nearly a million premature cancer deaths occurred between 1986 and 2004.
Accident

On 26 April 1986, at 01:23 ( UTC+3), reactor four suffered a catastrophic power increase, leading to explosions in its core. This dispersed large quantities of radioactive fuel and core materials into the atmosphere and ignited the combustible graphite moderator. The burning graphite moderator increased the emission of radioactive particles, carried by the smoke, as the reactor had not been encased by any kind of hard containment vessel. The accident occurred during an experiment scheduled to test a potential safety emergency core cooling feature, which took place during a normal shutdown procedure.
Steam turbine tests
An inactive nuclear reactor continues to generate a significant amount of residual decay heat. In an initial shut-down state (for example, following an emergency SCRAM) the reactor produces around 7 percent of its total thermal output and requires cooling to avoid core damage. RBMK reactors, like those at Chernobyl, use water as coolant. Reactor 4 at Chernobyl consisted of about 1,600 individual fuel channels; each required a coolant flow of 28 metric tons (28,000 liters / 7,400 US gallons) per hour.
Since cooling pumps require electricity to cool a reactor after a SCRAM, in the event of a power grid failure, Chernobyl's reactors had three backup diesel generators; these could start up in 15 seconds, but took 60–75 seconds to attain full speed and reach the 5.5‑ megawatt (MW) output required to run one main pump.
To solve this one-minute gap, considered an unacceptable safety risk, it had been theorised that rotational energy from the steam turbine (as it wound down under residual steam pressure) could be used to generate the required electrical power. Analysis indicated that this residual momentum and steam pressure might be sufficient to run the coolant pumps for 45 seconds, bridging the gap between an external power failure and the full availability of the emergency generators.
This capability still needed to be confirmed experimentally, and previous tests had ended unsuccessfully. An initial test carried out in 1982 showed that the excitation voltage of the turbine-generator was insufficient; it did not maintain the desired magnetic field after the turbine trip. The system was modified, and the test was repeated in 1984 but again proved unsuccessful. In 1985, the tests were attempted a third time but also yielded negative results. The test procedure was to be repeated again in 1986, and it was scheduled to take place during the maintenance shutdown of Reactor Four.
The test focused on the switching sequences of the electrical supplies for the reactor. The test procedure was to begin with an automatic emergency shutdown. No detrimental effect on the safety of the reactor was anticipated, so the test program was not formally coordinated with either the chief designer of the reactor (NIKIET) or the scientific manager. Instead, it was approved only by the director of the plant (and even this approval was not consistent with established procedures).
According to the test parameters, the thermal output of the reactor should have been no lower than 700 MW at the start of the experiment. If test conditions had been as planned, the procedure would almost certainly have been carried out safely; the eventual disaster resulted from attempts to boost the reactor output once the experiment had been started, which was inconsistent with approved procedure.
The Chernobyl power plant had been in operation for two years without the capability to ride through the first 60–75 seconds of a total loss of electric power, and thus lacked an important safety feature. The station managers presumably wished to correct this at the first opportunity, which may explain why they continued the test even when serious problems arose, and why the requisite approval for the test had not been sought from the Soviet nuclear oversight regulator (even though there was a representative at the complex of 4 reactors).
The experimental procedure was intended to run as follows:
- The reactor was to be running at a low power level, between 700 MW and 800 MW.
- The steam-turbine generator was to be run up to full speed.
- When these conditions were achieved, the steam supply for the turbine generator was to be closed off.
- Turbine generator performance was to be recorded to determine whether it could provide the bridging power for coolant pumps until the emergency diesel generators were sequenced to start and provide power to the cooling pumps automatically.
- After the emergency generators reached normal operating speed and voltage, the turbine generator would be allowed to freewheel down.
Conditions prior to the accident
The conditions to run the test were established before the day shift of 25 April 1986. The day shift workers had been instructed in advance and were familiar with the established procedures. A special team of electrical engineers was present to test the new voltage regulating system. As planned, a gradual reduction in the output of the power unit was begun at 01:06 on 25 April, and the power level had reached 50% of its nominal 3200 MW thermal level by the beginning of the day shift.
At this point, another regional power station unexpectedly went offline, and the Kiev electrical grid controller requested that the further reduction of Chernobyl's output be postponed, as power was needed to satisfy the peak evening demand. The Chernobyl plant director agreed, and postponed the test.
At 23:04, the Kiev grid controller allowed the reactor shut-down to resume. This delay had some serious consequences: the day shift had long since departed, the evening shift was also preparing to leave, and the night shift would not take over until midnight, well into the job. According to plan, the test should have been finished during the day shift, and the night shift would only have had to maintain decay heat cooling systems in an otherwise shut down plant.
The night shift had very limited time to prepare for and carry out the experiment. A further rapid reduction in the power level from 50% was executed during the shift change-over. Alexander Akimov was chief of the night shift, and Leonid Toptunov was the operator responsible for the reactor's operational regimen, including the movement of the control rods. Toptunov was a young engineer who had worked independently as a senior engineer for approximately three months.
The test plan called for a gradual reduction in power output from reactor 4 to a thermal level of 700–1000 MW. An output of 700 MW was reached at 00:05 on 26 April. However, due to the natural production of xenon-135, a neutron absorber, core power continued to decrease without further operator action—a process known as reactor poisoning. As the reactor power output dropped further, to approximately 500 MW, Toptunov mistakenly inserted the control rods too far—the exact circumstances leading to this are unknown because both Akimov and Toptunov were killed during the later explosion. This combination of factors rendered the reactor in an unintended near- shutdown state, with a power output of 30 MW thermal or less.
The reactor was now only producing around 5 percent of the minimum initial power level established as safe for the test. Control-room personnel consequently made the decision to restore power by extracting the majority of the reactor control rods to their upper limits. Several minutes elapsed between their extraction and the point that the power output began to increase and subsequently stabilize at 160–200 MW (thermal), a much smaller value than the planned 700 MW. The rapid reduction in the power during the initial shutdown, and the subsequent operation at a level of less than 200 MW led to increased poisoning of the reactor core by the accumulation of xenon-135. This restricted any further rise of reactor power, and made it necessary to extract additional control rods from the reactor core in order to counteract the poisoning.
The operation of the reactor at the low power level and high poisoning level, was accompanied by unstable core temperature and coolant flow, and possibly by instability of neutron flux. Various alarms started going off at this point. The control room received repeated emergency signals regarding the levels in the steam/water separator drums, and large excursions or variations in the flow rate of feed water, as well as from relief valves opened to relieve excess steam into a turbine condenser, and from the neutron power controller. In the period between 00:35 and 00:45, emergency alarm signals concerning thermal-hydraulic parameters were ignored, apparently to preserve the reactor power level. Emergency signals from the reactor emergency protection system (EPS-5) triggered a trip that turned off both turbine-generators.
After a while, a more or less stable state at a power level of 200 MW was achieved, and preparation for the experiment continued. As part of the test plan, extra water pumps were activated at 01:05 on 26 April, increasing the water flow. The increased coolant flow rate through the reactor produced an increase in the inlet coolant temperature of the reactor core, which now more closely approached the nucleate boiling temperature of water, reducing the safety margin.
The flow exceeded the allowed limit at 01:19. At the same time, the extra water flow lowered the overall core temperature and reduced the existing steam voids in the core. Since water also absorbs neutrons (and the higher density of liquid water makes it a better absorber than steam), turning on additional pumps decreased the reactor power further still. This prompted the operators to remove the manual control rods further to maintain power.
All these actions led to an extremely unstable reactor configuration. Nearly all of the control rods were removed, which would limit the value of the safety rods when initially inserted in a SCRAM condition. Further, the reactor coolant had reduced boiling, but had limited margin to boiling, so any power excursion would produce boiling, reducing neutron absorption by the water. The reactor was in an unstable configuration that was clearly outside the safe operating envelope established by the designers.
Experiment and explosion
At 1:23:04 a.m. the experiment began. Four (of eight total) Main Circulating Pumps (MCP) were active. The steam to the turbines was shut off, and a run down of the turbine generator began. The diesel generator started and sequentially picked up loads, which was complete by 01:23:43. During this period, the power for the four MCPs was supplied by the turbine generator as it coasted down. As the momentum of the turbine generator decreased, the water flow rate decreased, leading to increased formation of steam voids (bubbles) in the core.
Because of the positive void coefficient of the RBMK reactor at low reactor power levels, it was now primed to embark on a positive feedback loop, in which the formation of steam voids reduced the ability of the liquid water coolant to absorb neutrons, which in turn increased the reactor's power output. This caused yet more water to flash into steam, giving yet a further power increase. However, during almost the entire period of the experiment the automatic control system successfully counteracted this positive feedback, continuously inserting control rods into the reactor core to limit the power rise.
At 1:23:40, as recorded by the SKALA centralized control system, an emergency shutdown of the reactor, which inadvertently triggered the explosion, was initiated. The SCRAM was started when the EPS-5 button (also known as the AZ-5 button) of the reactor emergency protection system was pressed: this fully inserted all control rods, including the manual control rods that had been incautiously withdrawn earlier. The reason why the EPS-5 button was pressed is not known, whether it was done as an emergency measure or simply as a routine method of shutting down the reactor upon completion of the experiment.
There is a view that the SCRAM may have been ordered as a response to the unexpected rapid power increase, although there is no recorded data conclusively proving this. Some have suggested that the button was not pressed, and instead the signal was automatically produced by the emergency protection system; however, the SKALA clearly registered a manual SCRAM signal. In spite of this, the question as to when or even whether the EPS-5 button was pressed has been the subject of debate. There are assertions that the pressure was caused by the rapid power acceleration at the start, and allegations that the button was not pressed until the reactor began to self-destruct but others assert that it happened earlier and in calm conditions.
After the EPS-5 button was pressed, the insertion of control rods into the reactor core began. The control rod insertion mechanism moved the rods at 0.4 m/s, so that the rods took 18 to 20 seconds to travel the full height of the core, about 7 meters. A bigger problem was a flawed graphite-tip control rod design, which initially displaced coolant before inserting neutron-absorbing material to slow the reaction. As a result, the SCRAM actually increased the reaction rate in the lower half of the core.
A few seconds after the start of the SCRAM, a massive power spike occurred, the core overheated, and seconds later this overheating resulted in the initial explosion. Some of the fuel rods fractured, blocking the control rod columns and causing the control rods to become stuck at one-third insertion. Within three seconds the reactor output rose above 530 MW.
The subsequent course of events was not registered by instruments: it is known only as a result of mathematical simulation. Apparently, a great rise in power first caused an increase in fuel temperature and massive steam buildup, leading to a rapid increase in steam pressure. This destroyed fuel elements and ruptured the channels in which these elements were located.
Then, according to some estimations, the reactor jumped to around 30 GW thermal, ten times the normal operational output. The last reading on the control panel was 33 GW. It was not possible to reconstruct the precise sequence of the processes that led to the destruction of the reactor and the power unit building, but a steam explosion, like the explosion of a steam boiler from excess vapor pressure, appears to have been the next event. There is a general understanding that it was steam from the wrecked channels entering the reactor's inner structure that caused the destruction of the reactor casing, tearing off and lifting the 2,000-ton upper plate, to which the entire reactor assembly is fastened. Apparently, this was the first explosion that many heard. This explosion ruptured further fuel channels, and as a result the remaining coolant flashed to steam and escaped the reactor core. The total water loss in combination with a high positive void coefficient further increased the reactor power.
A second, more powerful explosion occurred about two or three seconds after the first; evidence indicates that the second explosion was from the core itself undergoing runaway criticality. The nuclear excursion dispersed the core and effectively terminated the nuclear chain reaction. However, a graphite fire was burning by now, greatly contributing to the spread of radioactive material and the contamination of outlying areas.
There were initially several hypotheses about the nature of the second explosion. One view was, "the second explosion was caused by the hydrogen which had been produced either by the overheated steam- zirconium reaction or by the reaction of red-hot graphite with steam that produced hydrogen and carbon monoxide." Another hypothesis was that the second explosion was a thermal explosion of the reactor as a result of the uncontrollable escape of fast neutrons caused by the complete water loss in the reactor core. A third hypothesis was that the explosion was caused by steam. According to this version, the flow of steam and the steam pressure caused all the destruction that followed the ejection from the shaft of a substantial part of the graphite and fuel.
According to observers outside Unit 4, burning lumps of material and sparks shot into the air above the reactor. Some of them fell on to the roof of the machine hall and started a fire. About 25 percent of the red-hot graphite blocks and overheated material from the fuel channels was ejected.... Parts of the graphite blocks and fuel channels were out of the reactor building.... As a result of the damage to the building an airflow through the core was established by the high temperature of the core. The air ignited the hot graphite and started a graphite fire.
However, the ratio of xenon radioisotopes released during the event indicates that the second explosion could be a nuclear power transient. This nuclear transient released 40 GJ of energy, the equivalent of about ten tons of TNT. The analysis indicates that the nuclear excursion was limited to a small portion of the core.
Contrary to safety regulations, bitumen, a combustible material, had been used in the construction of the roof of the reactor building and the turbine hall. Ejected material ignited at least five fires on the roof of the adjacent reactor 3, which was still operating. It was imperative to put those fires out and protect the cooling systems of reactor 3. Inside reactor 3, the chief of the night shift, Yuri Bagdasarov, wanted to shut down the reactor immediately, but chief engineer Nikolai Fomin would not allow this. The operators were given respirators and potassium iodide tablets and told to continue working. At 05:00, however, Bagdasarov made his own decision to shut down the reactor, leaving only those operators there who had to work the emergency cooling systems.
Radiation levels
Approximate radiation levels at different locations shortly after the explosion were as follows:
Location | Radiation ( Roentgens per hour) | Sieverts per hour (SI Unit) |
---|---|---|
Vicinity of the reactor core | 30,000 | 300 |
Fuel fragments | 15,000–20,000 | 150–200 |
Debris heap at the place of circulation pumps | 10,000 | 100 |
Debris near the electrolyzers | 5,000–15,000 | 50–150 |
Water in the Level +25 feedwater room | 5,000 | 50 |
Level 0 of the turbine hall | 500–15,000 | 5–150 |
Area of the affected unit | 1,000–1,500 | 10–15 |
Water in Room 712 | 1,000 | 10 |
Control room | 3–5 | 0.03–0.05 |
Gidroelektromontazh depot | 30 | 0.3 |
Nearby concrete mixing unit | 10–15 | 0.10–0.15 |
Plant layout
- Based on the image of the plant
Level | Objects |
---|---|
Metres | Levels are distances above (or below for minus values) ground level at the site. |
49.6 | Roof of the reactor building, gallery of the refueling mechanism |
39.9 | Roof of the deaerator gallery |
35.5 | Floor of the main reactor hall |
31.6 | Upper side of the upper biological shield, floor of the space for pipes to steam separators |
28.3 | Lower side of the turbine hall roof |
24.0 | Deaerator floor, measurement and control instruments room |
16.4 | Floor of the pipe aisle in the deaerator gallery |
12.0 | Main floor of the turbine hall, floor of the main circulation pump motor compartments |
10.0 | Control room, floor under the reactor lower biological shield, main circulation pumps |
6.0 | Steam distribution corridor |
2.2 | Upper pressure suppression pool |
0.0 | Ground level; house switchgear, turbine hall level |
−0.5 | Lower pressure suppression pool |
−5.2, −4.2 | Other turbine hall levels |
−6.5 | Basement floor of the turbine hall |
Individual involvement
Causes
Operator error
There were two official explanations of the accident: the first, later acknowledged to be erroneous, was published in August 1986 and effectively placed the blame on the power plant operators. To investigate the causes of the accident the IAEA created a group known as the International Nuclear Safety Advisory Group (INSAG), which in its report of 1986, INSAG-1, on the whole also supported this view, based on the data provided by the Soviets and the oral statements of specialists. In this view, the catastrophic accident was caused by gross violations of operating rules and regulations. "During preparation and testing of the turbine generator under run-down conditions using the auxiliary load, personnel disconnected a series of technical protection systems and breached the most important operational safety provisions for conducting a technical exercise."
The operator error was probably due to their lack of knowledge of nuclear reactor physics and engineering, as well as lack of experience and training. According to these allegations, at the time of the accident the reactor was being operated with many key safety systems turned off, most notably the Emergency Core Cooling System (ECCS), LAR (Local Automatic control system), and AZ (emergency power reduction system). Personnel had an insufficiently detailed understanding of technical procedures involved with the nuclear reactor, and knowingly ignored regulations to speed test completion.
The developers of the reactor plant considered this combination of events to be impossible and therefore did not allow for the creation of emergency protection systems capable of preventing the combination of events that led to the crisis, namely the intentional disabling of emergency protection equipment plus the violation of operating procedures. Thus the primary cause of the accident was the extremely improbable combination of rule infringement plus the operational routine allowed by the power station staff.
In this analysis of the causes of the accident, deficiencies in the reactor design and in the operating regulations that made the accident possible were set aside and mentioned only casually. Serious critical observations covered only general questions and did not address the specific reasons for the accident. The following general picture arose from these observations. Several procedural irregularities also helped to make the accident possible. One was insufficient communication between the safety officers and the operators in charge of the experiment being run that night.
The reactor operators disabled safety systems down to the generators, which the test was really about. The main process computer, SKALA, was running in such a way that the main control computer could not shut down the reactor or even reduce power. Normally the reactor would have started to insert all of the control rods. The computer would have also started the "Emergency Core Protection System" that introduces 24 control rods into the active zone within 2.5 seconds, which is still slow by 1986 standards. All control was transferred from the process computer to the human operators.
On the subject of the disconnection of safety systems, Valery Legasov said, in 1987, "It was like airplane pilots experimenting with the engines in flight."
This view is reflected in numerous publications and also artistic works on the theme of the Chernobyl accident that appeared immediately after the accident, and for a long time remained dominant in the public consciousness and in popular publications.
Operating instructions and design deficiencies found
In 1991 a Commission of the USSR State Committee for the Supervision of Safety in Industry and Nuclear Power has reassessed the causes and circumstances of the Chernobyl accident and came to new insights and conclusions. Based on it, in 1992 the IAEA Nuclear Safety Advisory Group (INSAG) published an additional report, INSAG-7, which reviewed "that part of the INSAG-1 report in which primary attention is given to the reasons for the accident". and included the USSR State Commission report as Appendix I.
In this INSAG report, most of the earlier accusations against staff for breach of regulations were acknowledged to be either erroneous, based on incorrect information obtained in August 1986, or less relevant. This report reflected another view of the main reasons for the accident, presented in Appendix I. According to this account, the operators' actions in turning off the Emergency Core Cooling System, interfering with the settings on the protection equipment, and blocking the level and pressure in the separator drum did not contribute to the original cause of the accident and its magnitude, although they may have been a breach of regulations. Turning off the emergency system designed to prevent the two turbine generators from stopping was not a violation of regulations.
Human factors contributed to the conditions that led to the disaster. These included operating the reactor at a low power level – less than 700 MW – a level documented in the run-down test program, and operating with a small operational reactivity margin (ORM). The 1986 assertions of Soviet experts notwithstanding, regulations did not prohibit operating the reactor at this low power level.
However, regulations did forbid operating the reactor with a small margin of reactivity. Yet "post-accident studies have shown that the way in which the real role of the ORM is reflected in the Operating Procedures and design documentation for the RBMK-1000 is extremely contradictory," and furthermore, "ORM was not treated as an operational safety limit, violation of which could lead to an accident."
According to the INSAG-7 Report, the chief reasons for the accident lie in the peculiarities of physics and in the construction of the reactor. There are two such reasons:
- The reactor had a dangerously large positive void coefficient. The void coefficient is a measurement of how a reactor responds to increased steam formation in the water coolant. Most other reactor designs have a negative coefficient, i.e. the nuclear reaction rate slows when steam bubbles form in the coolant, since as the vapor phase in the reactor increases, fewer neutrons are slowed down. Faster neutrons are less likely to split uranium atoms, so the reactor produces less power (a negative feed-back). Chernobyl's RBMK reactor, however, used solid graphite as a neutron moderator to slow down the neutrons, and the water in it, on the contrary, acts like a harmful neutron absorber. Thus neutrons are slowed down even if steam bubbles form in the water. Furthermore, because steam absorbs neutrons much less readily than water, increasing the intensity of vaporization means that more neutrons are able to split uranium atoms, increasing the reactor's power output. This makes the RBMK design very unstable at low power levels, and prone to suddenly increasing energy production to a dangerous level. This behaviour is counter-intuitive, and this property of the reactor was unknown to the crew.
- A more significant flaw was in the design of the control rods that are inserted into the reactor to slow down the reaction. In the RBMK reactor design, the lower part of each control rod was made of graphite and was 1.3 meters shorter than necessary, and in the space beneath the rods were hollow channels filled with water. The upper part of the rod, the truly functional part that absorbs the neutrons and thereby halts the reaction, was made of boron carbide. With this design, when the rods are inserted into the reactor from the uppermost position, the graphite parts initially displace some water (which absorbs neutrons, as mentioned above), effectively causing less neutrons to be absorbed initially. Thus for the first few seconds of control rod activation, reactor power output is increased, rather than reduced as desired. This behaviour is counter-intuitive and was not known to the reactor operators.
- Other deficiencies besides these were noted in the RBMK-1000 reactor design, as were its non-compliance with accepted standards and with the requirements of nuclear reactor safety.
Both views were heavily lobbied by different groups, including the reactor's designers, power plant personnel, and the Soviet and Ukrainian governments. According to the IAEA's 1986 analysis, the main cause of the accident was the operators' actions. But according to the IAEA's 1993 revised analysis the main cause was the reactor's design. One reason there were such contradictory viewpoints and so much debate about the causes of the Chernobyl accident was that the primary data covering the disaster, as registered by the instruments and sensors, were not completely published in the official sources.
Once again, the human factor had to be considered as a major element in causing the accident. INSAG notes that both the operating regulations and staff handled the disabling of the reactor protection easily enough: witness the length of time for which the ECCS was out of service while the reactor was operated at half power. INSAG's view is that it was the operating crew's deviation from the test program that was mostly to blame. "Most reprehensibly, unapproved changes in the test procedure were deliberately made on the spot, although the plant was known to be in a very different condition from that intended for the test."
As in the previously released report INSAG-1, close attention is paid in report INSAG-7 to the inadequate (at the moment of the accident) "culture of safety" at all levels. Deficiency in the safety culture was inherent not only at the operational stage but also, and to no lesser extent, during activities at other stages in the lifetime of nuclear power plants (including design, engineering, construction, manufacture and regulation). The poor quality of operating procedures and instructions, and their conflicting character, put a heavy burden on the operating crew, including the Chief Engineer. "The accident can be said to have flowed from a deficient safety culture, not only at the Chernobyl plant, but throughout the Soviet design, operating and regulatory organizations for nuclear power that existed at that time."
Effects
International spread of radioactive substances

Four hundred times more radioactive material was released than had been by the atomic bombing of Hiroshima. The disaster released 1/100 to 1/1000 of the total amount of radioactivity released by nuclear weapons testing during the 1950s and 1960s. Approximately 100,000 km² of land was significantly contaminated with fallout, the worst hit regions being in Belarus, Ukraine and Russia. Slighter levels of contamination were detected over all of Europe except for the Iberian Peninsula.
The initial evidence that a major release of radioactive material was affecting other countries came not from Soviet sources, but from Sweden, where on the morning of 28 April workers at the Forsmark Nuclear Power Plant(approximately 1,100 km (680 mi) from the Chernobyl site) were found to have radioactive particles on their clothes.
It was Sweden's search for the source of radioactivity, after they had determined there was no leak at the Swedish plant, that at noon on 28 April led to the first hint of a serious nuclear problem in the western Soviet Union. Hence the evacuation of Pripyat on 27 April 36 hours after the initial explosions, was silently completed before the disaster became known outside the Soviet Union. The rise in radiation levels had at that time already been measured in Finland, but a civil service strike delayed the response and publication.
Country | 37–185 k Bq/m2 | 185–555 kBq/m2 | 555–1480 kBq/m2 | >1480 kBq/m2 | ||||
---|---|---|---|---|---|---|---|---|
km2 | % of country | km2 | % of country | km2 | % of country | km2 | % of country | |
Belarus | 29,900 | 14.4 | 10,200 | 4.9 | 4,200 | 2.0 | 2,200 | 1.1 |
Ukraine | 37,200 | 6.2 | 3,200 | 0.53 | 900 | 0.15 | 600 | 0.1 |
Russia | 49,800 | 0.29 | 5,700 | 0.03 | 2,100 | 0.01 | 300 | 0.002 |
Sweden | 12,000 | 2.7 | — | — | — | — | — | — |
Finland | 11,500 | 3.4 | — | — | — | — | — | — |
Austria | 8,600 | 10.3 | — | — | — | — | — | — |
Norway | 5,200 | 1.3 | — | — | — | — | — | — |
Bulgaria | 4,800 | 4.3 | — | — | — | — | — | — |
Switzerland | 1,300 | 3.1 | — | — | — | — | — | — |
Greece | 1,200 | 0.91 | — | — | — | — | — | — |
Slovenia | 300 | 1.5 | — | — | — | — | — | — |
Italy | 300 | 0.1 | — | — | — | — | — | — |
Moldova | 60 | 0.2 | — | — | — | — | — | — |
Totals | 162,160 km2 | 19,100 km2 | 7,200 km2 | 3,100 km2 |
Contamination from the Chernobyl accident was scattered irregularly depending on weather conditions, much of it deposited on mountainous regions such as the Alps, Wales and the Scottish Highlands, where adibatic cooling caused rainfall. The resulting patches of contamination could be highly localised, and water-flows across the ground contributed further to large variations in radioactivity over small areas. Sweden and Norway also received heavy fallout when the contaminated air collided with a cold front, bringing rain.
Rain was purposely seeded over 10,000 km2 of the Belorussian SSR by the Soviet air force to remove radioactive particles from clouds heading toward highly populated areas. Heavy, black-coloured rain fell on the city of Gomel. Reports from Soviet and Western scientists indicate that Belarus received about 60% of the contamination that fell on the former Soviet Union. However, the 2006 TORCH report stated that half of the volatile particles had landed outside Ukraine, Belarus, and Russia. A large area in Russia south of Bryansk was also contaminated, as were parts of northwestern Ukraine. Studies in surrounding countries indicate that over one million people could have been affected by radiation.
Recently published data from a long-term monitoring program (The Korma Report) shows a decrease in internal radiation exposure of the inhabitants of a region in Belarus close to Gomel. Resettlement may even be possible in prohibited areas provided that people comply with appropriate dietary rules.
In Western Europe, precautionary measures taken in response to the radiation included seemingly arbitrary regulations banning the importation of certain foods but not others. In France some officials stated that the Chernobyl accident had no adverse effects. Official figures in southern Bavaria in Germany indicated that some wild plant species contained substantial levels of caesium, which were believed to have been passed onto them by wild boars, a significant number of which had already contained radioactive particles above the allowed level, consuming them.
Radioactive release
Like many other releases of radioactivity into the environment, the Chernobyl release was controlled by the physical and chemical properties of the radioactive elements in the core. While the general population often perceives plutonium as a particularly dangerous nuclear fuel, its effects are almost eclipsed by those of its fission products. Particularly dangerous are highly radioactive compounds that accumulate in the food chain, such as some isotopes of iodine and strontium.
Two reports on the release of radioisotopes from the site were made available, one by the OSTI and a more detailed report by the OECD, both in 1998. At different times after the accident, different isotopes were responsible for the majority of the external dose. The dose that was calculated is that received from external gamma irradiation for a person standing in the open. The dose to a person in a shelter or the internal dose is harder to estimate.
The release of radioisotopes from the nuclear fuel was largely controlled by their boiling points, and the majority of the radioactivity present in the core was retained in the reactor.
- All of the noble gases, including krypton and xenon, contained within the reactor were released immediately into the atmosphere by the first steam explosion.
- 55% of the radioactive iodine in the reactor, containing about 1760 P Bq or 400 kg of I-131, was released, as a mixture of vapor, solid particles, and organic iodine compounds.
- Caesium (85 PBq Cs-137) and tellurium were released in aerosol form.
- An early estimate for fuel material released to the environment was 3 ± 1.5%; this was later revised to 3.5 ± 0.5%. This corresponds to the atmospheric emission of 6 t of fragmented fuel.
- Total atmospheric release is estimated at 5200 P Bq.
Two sizes of particles were released: small particles of 0.3 to 1.5 micrometers ( aerodynamic diameter) and large particles of 10 micrometers. The large particles contained about 80% to 90% of the released nonvolatile radioisotopes zirconium-95, niobium-95, lanthanum-140, cerium-144 and the transuranic elements, including neptunium, plutonium and the minor actinides, embedded in a uranium oxide matrix.
Health of plant workers and local people

In the aftermath of the accident, 237 people suffered from acute radiation sickness (ARS), of whom 31 died within the first three months. Most of the victims were fire and rescue workers trying to bring the accident under control, who were not fully aware of how dangerous the exposure to radiation in the smoke was. Whereas, in the World Health Organization's 2006 report of the Chernobyl Forum expert group on the 237 emergency workers who were diagnosed with ARS, ARS was identified as the cause of death for 28 of these people within the first few months after the disaster.
No further ARS-related deaths were identified in the general population affected by the disaster. Of the 72,000 Russian Emergency Workers being studied, 216 non-cancer deaths are attributed to the disaster, between 1991 and 1998. Of all 66,000 Belarusian emergency workers, by the mid-1990s only 150 (roughly 0.2%) were reported by their government as having died. In contrast, 5,722 casualties were reported among Ukrainian clean-up workers up to the year 1995, by the National Committee for Radiation Protection of the Ukrainian Population.
The latency period for solid cancers caused by excess radiation exposure is 10 or more years; thus at the time of the WHO report being undertaken, the rates of solid cancer deaths were no greater than the general population. Some 135,000 people were evacuated from the area, including 50,000 from Pripyat.
Residual radioactivity in the environment
Rivers, lakes and reservoirs
The Chernobyl nuclear power plant is located next to the Pripyat River, which feeds into the Dnieper reservoir system, one of the largest surface water systems in Europe, which at the time supplied water to Kiev's 2.4 million residents, and was still in spring flood when the accident occurred. The radioactive contamination of aquatic systems therefore became a major problem in the immediate aftermath of the accident. In the most affected areas of Ukraine, levels of radioactivity (particularly from radionuclides 131I, 137Cs and 90Sr) in drinking water caused concern during the weeks and months after the accident, though officially it was stated that all contaminants had settled to the bottom "in an insoluble phase" and would not dissolve for 800–1,000 years. Guidelines for levels of radioiodine in drinking water were temporarily raised to 3,700 Bq/L, allowing most water to be reported as safe, and a year after the accident it was announced that even the water of the Chernobyl plant's cooling pond was within acceptable norms. Despite this, two months after the disaster the Kiev water supply was abruptly switched from the Dnieper to the Desna River. Meanwhile, massive silt traps were constructed, along with an enormous 30m-deep underground barrier to prevent groundwater from the destroyed reactor entering the Pripyat River.
Bio-accumulation of radioactivity in fish resulted in concentrations (both in western Europe and in the former Soviet Union) that in many cases were significantly above guideline maximum levels for consumption. Guideline maximum levels for radiocaesium in fish vary from country to country but are approximately 1,000 Bq/kg in the European Union. In the Kiev Reservoir in Ukraine, concentrations in fish were several thousand Bq/kg during the years after the accident.
In small "closed" lakes in Belarus and the Bryansk region of Russia, concentrations in a number of fish species varied from 100 to 60,000 Bq/kg during the period 1990–92. The contamination of fish caused short-term concern in parts of the UK and Germany and in the long term (years rather than months) in the affected areas of Ukraine, Belarus, and Russia as well as in parts of Scandinavia.
Groundwater
Groundwater was not badly affected by the Chernobyl accident since radionuclides with short half-lives decayed away long before they could affect groundwater supplies, and longer-lived radionuclides such as radiocaesium and radiostrontium were adsorbed to surface soils before they could transfer to groundwater. However, significant transfers of radionuclides to groundwater have occurred from waste disposal sites in the 30 km (19 mi) exclusion zone around Chernobyl. Although there is a potential for transfer of radionuclides from these disposal sites off-site (i.e. out of the 30 km (19 mi) exclusion zone), the IAEA Chernobyl Report argues that this is not significant in comparison to current levels of washout of surface-deposited radioactivity.
Flora and fauna

After the disaster, four square kilometers of pine forest directly downwind of the reactor turned reddish-brown and died, earning the name of the " Red Forest". Some animals in the worst-hit areas also died or stopped reproducing. Most domestic animals were removed from the exclusion zone, but horses left on an island in the Pripyat River 6 km (4 mi) from the power plant died when their thyroid glands were destroyed by radiation doses of 150–200 Sv. Some cattle on the same island died and those that survived were stunted because of thyroid damage. The next generation appeared to be normal.
A robot sent into the reactor itself has returned with samples of black, melanin-rich radiotrophic fungi that are growing on the reactor's walls.
Of the 440,350 wild boar killed in the 2010 hunting season in Germany, over 1,000 were found to be contaminated with levels of radiation above the permitted limit of 600 bequerels, due to residual radioactivity from Chernobyl. Germany has "banned wild game meat because of contamination linked to radioactive mushrooms".
The Norwegian Agricultural Authority reported that in 2009 a total of 18,000 livestock in Norway needed to be given uncontaminated feed for a period of time before slaughter in order to ensure that their meat was safe for human consumption. This was due to residual radioactivity from Chernobyl in the plants they graze on in the wild during the summer. The after-effects of Chernobyl were expected to be seen for a further 100 years, although the severity of the effects would decline over that period. In Britain and Norway, as of 2011, "slaughter restrictions remain for sheep raised on pasture contaminated by radiation fallout".
Human impact

The Chernobyl Forum first met on 3 February 2003 for a three day meeting. It consisted of the International Atomic Energy Agency (IAEA), other United Nations organizations (FAO, UN-OCHA, UNDP, UNEP, UNSCEAR, WHO, and the World Bank), and the governments of Belarus, Russia, and Ukraine. A second meeting was held on 10–11 March 2004, and a third on 18–20 April 2005. The aim of the Forum was to "scientifically clarify the radiological environmental and health consequences of the Chernobyl accident, to provide advice on and to contribute to a scientifically sound remediation and health care programmes, and to consider the necessity of, and opportunities for continued research/learning lessons."
Thyroid cancer
A report was published by Chernobyl Forum in 2005 that revealed thyroid cancer among children to be one of the main health impacts from the Chornobyl accident. In that publication more than 4000 cases were reported, and that there was no evidence of an increase in solid cancers or leukemia. It said that there was an increase in psychological problems among the affected population. The report says it is impossible to reliably predict the number of fatal cancers arising from the incident as small differences in assumptions can result in large differences in the estimated health costs. The report says it represents the consensus view of the eight UN organisations.
On the death toll of the accident, the report states that twenty-eight emergency workers ("liquidators") died from acute radiation syndrome including beta burns and 15 patients died from thyroid cancer in the following years, and it roughly estimated that cancer deaths caused by Chernobyl may reach a total of about 4,000 among the 5 million persons residing in the contaminated areas, the report projected cancer mortality "increases of less than one per cent" (~0.3%) on a time span of 80 years, cautioning that this estimate was "speculative" since at this time only a few tens of cancer deaths are linked to the Chernobyl disaster.
According to UNSCEAR, up to the year 2005 more than 6000 cases of thyroid cancer were reported in children and adolescents exposed at the time of the accident, a number that is expected to increase. They concluded that was no other evidence of major health impacts from the radiation exposure.
Well-differentiated thyroid cancers are generally treatable, and when treated the five-year survival rate of thyroid cancer is 96%, and 92% after 30 years. UNSCEAR had reported 15 deaths from thyroid cancer in 2011. The International Atomic Energy Agency (IAEA) also states that there has been no increase in the rate of birth defects or abnormalities, or solid cancers (such as lung cancer) corroborating UNSCEAR's assessments. UNSCEAR does raise the possibility of long term genetic defects, pointing to a doubling of radiation-induced minisatellite mutations among children born in 1994. However, the risk of thyroid cancer associated with the Chernobyl accident is still high according to published studies.
Other health disorders
Fred Mettler, a radiation expert at the University of New Mexico, puts the number of worldwide cancer deaths outside the highly contaminated zone at "perhaps" 5000, for a total of 9000 Chernobyl-associated fatal cancers, saying "the number is small (representing a few percent) relative to the normal spontaneous risk of cancer, but the numbers are large in absolute terms". The same report outlined studies based in data found in the Russian Registry from 1991 to 1998 that suggested that "of 61,000 Russian workers exposed to an average dose of 107 mSv about 5% of all fatalities that occurred may have been due to radiation exposure."
The report went into depth about the risks to mental health of exaggerated fears about the effects of radiation. According to the IAEA the "designation of the affected population as "victims" rather than "survivors" has led them to perceive themselves as helpless, weak and lacking control over their future". The IAEA says that this may have led to behaviour that has caused further health effects.
Fred Mettler commented that 20 years later "The population remains largely unsure of what the effects of radiation actually are and retain a sense of foreboding. A number of adolescents and young adults who have been exposed to modest or small amounts of radiation feel that they are somehow fatally flawed and there is no downside to using illicit drugs or having unprotected sex. To reverse such attitudes and behaviors will likely take years although some youth groups have begun programs that have promise." In addition, disadvantaged children around Chernobyl suffer from health problems that are attributable not only to the Chernobyl accident, but also to the poor state of post-Soviet health systems.
The United Nations Scientific Committee on the Effects of Atomic Radiation (UNSCEAR), part of the Chernobyl Forum, have produced their own assessments of the radiation effects. UNSCEAR was set up as a collaboration between various United Nation bodies, including the World Health Organisation, after the atomic bomb attacks on Hiroshima and Nagasaki, to assess the long-term effects of radiation on human health.
Deaths due to radiation exposure
The number potential deaths arising from the Chernobyl disaster is heavily debated. The WHO's prediction of 4,000 future cancer deaths in surrounding countries is based on the Linear no-threshold model (LNT), which assumes that the damage inflicted by radiation at low doses is directly proportional to the dose. Radiation epidemiologist Roy Shore contends that estimating health effects in a population from the LNT model "is not wise because of the uncertainties".
According to the Union of Concerned Scientists the number of excess cancer deaths worldwide (including all contaminated areas) is approximately 27,000 based on the same LNT.
Another study critical of the Chernobyl Forum report was commissioned by Greenpeace, which asserts that "the most recently published figures indicate that in Belarus, Russia and Ukraine alone the accident could have resulted in an estimated 200,000 additional deaths in the period between 1990 and 2004." The Scientific Secretary of the Chernobyl Forum criticized the report's exclusive reliance on non- peer reviewed locally produced studies (in fact, most of the study's sources are from peer-reviewed journals, including many Western medical journals, or from proceedings of scientific conferences), while Gregory Härtl (spokesman for the WHO) suggested that the conclusions were motivated by ideology.
The German affiliate of the International Physicians for the Prevention of Nuclear War (IPPNW) argued that more than 10,000 people are today affected by thyroid cancer and 50,000 cases are expected in the future.
Chernobyl: Consequences of the Catastrophe for People and the Environment is an English translation of the 2007 Russian publication Chernobyl. It was published in 2009 by the New York Academy of Sciences in their Annals of the New York Academy of Sciences. It presents an analysis of scientific literature and concludes that medical records between 1986, the year of the accident, and 2004 reflect 985,000 premature deaths as a result of the radioactivity released.
The authors suggest that most of the deaths were in Russia, Belarus and Ukraine, though others occurred worldwide throughout the many countries that were struck by radioactive fallout from Chernobyl. The literature analysis draws on over 1,000 published titles and over 5,000 internet and printed publications discussing the consequences of the Chernobyl disaster. The authors contend that those publications and papers were written by leading Eastern European authorities and have largely been downplayed or ignored by the IAEA and UNSCEAR. This estimate has however been criticized as exaggerated, lacking a proper scientific base.
Other conditions
According to Kenneth Mossman, a Professor of Health Physics and member of the U.S. Nuclear Regulatory Commission advisory committee, the "LNT philosophy is overly conservative, and low-level radiation may be less dangerous than commonly believed". Yoshihisa Matsumoto, a radiation biologist at the Tokyo Institute of Technology, cites laboratory experiments on animals to suggest there must be a threshold dose below which DNA repair mechanisms can completely repair any radiation damage. Mossman suggests that the proponents of the current model believe that being conservative is justified due to the uncertainties surrounding low level doses and it is better to have a "prudent public health policy".
Another significant issue is establishing consistent data on which to base the analysis of the impact of the Chernobyl accident. Since 1991 large social and political changes have occurred within the affected regions and these changes have had significant impact on the administration of health care, on socio-economic stability, and the manner in which statistical data is collected. Ronald Chesser, a radiation biologist at Texas Tech University, says that "the subsequent Soviet collapse, scarce funding, imprecise dosimetry, and difficulties tracking people over the years have limited the number of studies and their reliability."
Economic and political consequences
It is difficult to establish the total economic cost of the disaster. According to Mikhail Gorbachev, the Soviet Union spent 18 billion rubles (the equivalent of US$18 billion at that time) on containment and decontamination, virtually bankrupting itself. In Belarus the total cost over 30 years is estimated at US$235 billion (in 2005 dollars). On-going costs are well known; in their 2003–2005 report, The Chernobyl Forum stated that between 5% and 7% of government spending in Ukraine still related to Chernobyl, while in Belarus over $13 billion is thought to have been spent between 1991 and 2003, with 22% of national budget having been Chernobyl-related in 1991, falling to 6% by 2002. Much of the current cost relates to the payment of Chernobyl-related social benefits to some 7 million people across the 3 countries.
A significant economic impact at the time was the removal of 784,320 ha (1,938,100 acres) of agricultural land and 694,200 ha (1,715,000 acres) of forest from production. While much of this has been returned to use, agricultural production costs have risen due to the need for special cultivation techniques, fertilizers and additives.
Politically, the accident gave great significance to the new Soviet policy of glasnost, and helped forge closer Soviet-US relations at the end of the Cold War, through bioscientific cooperation. But the disaster also became a key factor in the Union's eventual 1991 dissolution, and a major influence in shaping the new Eastern Europe.
Aftermath
Following the accident, questions arose about the future of the plant and its eventual fate. All work on the unfinished reactors 5 and 6 was halted three years later. However, the trouble at the Chernobyl plant did not end with the disaster in reactor 4. The damaged reactor was sealed off and 200 cubic meters (260 cu yd) of concrete was placed between the disaster site and the operational buildings. The Ukrainian government continued to let the three remaining reactors operate because of an energy shortage in the country.
Decommissioning
In 1991, a fire broke out in the turbine building of reactor 2; the authorities subsequently declared the reactor damaged beyond repair and had it taken offline. Reactor 1 was decommissioned in November 1996 as part of a deal between the Ukrainian government and international organizations such as the IAEA to end operations at the plant. On 15 December 2000, then-President Leonid Kuchma personally turned off Reactor 3 in an official ceremony, shutting down the entire site.
Radioactive waste management
Containment of the reactor
The Chernobyl reactor is now enclosed in a large concrete sarcophagus, which was built quickly to allow continuing operation of the other reactors at the plant.
A New Safe Confinement was to have been built by the end of 2005; however, it has suffered ongoing delays and as of 2010, when construction finally began, was expected to be completed in 2013, however was delayed again to 2016, the end of the 30-year lifespan of the sarcophagus. The structure is being built adjacent to the existing shelter and will be slid into place on rails. It is to be a metal arch 105 metres (344 ft) high and spanning 257 metres (843 ft), to cover both unit 4 and the hastily built 1986 structure. The Chernobyl Shelter Fund, set up in 1997, has received €810 million from international donors and projects to cover this project and previous work. It and the Nuclear Safety Account, also applied to Chernobyl decommissioning, are managed by the European Bank for Reconstruction and Development (EBRD).
By 2002, roughly 15,000 Ukrainian workers were still working within the Zone of Exclusion, maintaining the plant and performing other containment- and research-related tasks, often in dangerous conditions. A handful of Ukrainian scientists work inside the sarcophagus, but outsiders are rarely granted access. In 2006 an Australian 60 Minutes team led by reporter Richard Carleton and producer Stephen Rice were allowed to enter the sarcophagus for 15 minutes and film inside the control room.
Radioactive materials and waste management
As of 2006, some fuel remained in the reactors at units 1 through 3, most of it in each unit's cooling pond, as well as some material in a small spent fuel interim storage facility pond (ISF-1).
In 1999 a contract was signed for construction of a radioactive waste management facility to store 25,000 used fuel assemblies from units 1–3 and other operational wastes, as well as material from decommissioning units 1–3 (which will be the first RBMK units decommissioned anywhere). The contract included a processing facility able to cut the RBMK fuel assemblies and to put the material in canisters, which were to be filled with inert gas and welded shut.
The canisters were to be transported to dry storage vaults, where the fuel containers would be enclosed for up to 100 years. This facility, treating 2500 fuel assemblies per year, would be the first of its kind for RBMK fuel. However, after a significant part of the storage structures had been built, technical deficiencies in the concept emerged, and the contract was terminated in 2007. The interim spent fuel storage facility (ISF-2) will now be completed by others by mid-2013.
Another contract has been let for a liquid radioactive waste treatment plant, to handle some 35,000 cubic meters of low- and intermediate-level liquid wastes at the site. This will need to be solidified and eventually buried along with solid wastes on site.
In January 2008, the Ukrainian government announced a 4-stage decommissioning plan that incorporates the above waste activities and progresses towards a cleared site .
Lava-like fuel-containing materials (FCMs)
According to official estimates, about 95% of the fuel in the reactor at the time of the accident (about 180 metric tons) remains inside the shelter, with a total radioactivity of nearly 18 million curies (670 PBq). The radioactive material consists of core fragments, dust, and lava-like " fuel containing materials" (FCM, also called "corium") that flowed through the wrecked reactor building before hardening into a ceramic form.
Three different lavas are present in the basement of the reactor building: black, brown, and a porous ceramic. They are silicate glasses with inclusions of other materials within them. The porous lava is brown lava that dropped into water and thus cooled rapidly.
Degradation of the lava
It is unclear how long the ceramic form will retard the release of radioactivity. From 1997 to 2002 a series of papers were published that suggested that the self-irradiation of the lava would convert all 1,200 metric tons into a submicrometer and mobile powder within a few weeks. But it has been reported that the degradation of the lava is likely to be a slow and gradual process rather than sudden and rapid. The same paper states that the loss of uranium from the wrecked reactor is only 10 kg (22 lb) per year. This low rate of uranium leaching suggests that the lava is resisting its environment. The paper also states that when the shelter is improved, the leaching rate of the lava will decrease.
Some of the surfaces of the lava flows have started to show new uranium minerals such as Na4(UO2)(CO3)3 and uranyl carbonate. However, the level of radioactivity is such that during one hundred years the self irradiation of the lava (2 × 1016 α decays per gram and 2 to 5 × 105 Gy of β or γ) will fall short of the level of self irradiation required to greatly change the properties of glass (1018 α decays per gram and 108 to 109 Gy of β or γ). Also the rate of dissolution of the lava in water is very low (10−7 g-cm−2 day−1), suggesting that the lava is unlikely to dissolve in water.
The Exclusion Zone
An area extending 19 miles (31 km) in all directions from the plant is known as the "zone of alienation." It is largely uninhabited, except for a few residents who have refused to leave. The area has largely reverted to forest. Even today, radiation levels are so high that the workers responsible for rebuilding the sarcophagus are only allowed to work five hours a day for one month before taking 15 days of rest. Ukrainian officials estimate the area will not be safe for human life again for another 20,000 years.
In 2011, Ukraine opened up the sealed zone around the Chernobyl reactor to tourists who wish to learn more about the tragedy that occurred in 1986.
Recovery projects
The Chernobyl Shelter Fund
The Chernobyl Shelter Fund was established in 1997 at the Denver 23rd G8 summit to finance the Shelter Implementation Plan (SIP). The plan calls for transforming the site into an ecologically safe condition by means of stabilization of the sarcophagus followed by construction of a New Safe Confinement (NSC). While the original cost estimate for the SIP was US$768 million, the 2006 estimate was $1.2 billion. The SIP is being managed by a consortium of Bechtel, Battelle, and Electricité de France, and conceptual design for the NSC consists of a movable arch, constructed away from the shelter to avoid high radiation, to be slid over the sarcophagus. The NSC is expected to be completed in 2015, and will be the largest movable structure ever built.
Dimensions:
- Span: 270 m (886 ft)
- Height: 100 m (330 ft)
- Length: 150 m (492 ft)
The United Nations Development Programme
The United Nations Development Programme has launched in 2003 a specific project called the Chernobyl Recovery and Development Programme (CRDP) for the recovery of the affected areas. The programme was initiated in February 2002 based on the recommendations in the report on Human Consequences of the Chernobyl Nuclear Accident. The main goal of the CRDP's activities is supporting the Government of Ukraine in mitigating long-term social, economic, and ecological consequences of the Chernobyl catastrophe. CRDP works in the four most Chernobyl-affected areas in Ukraine: Kyivska, Zhytomyrska, Chernihivska and Rivnenska.
The International Project on the Health Effects of the Chernobyl Accident
The International Project on the Health Effects of the Chernobyl Accident (IPEHCA) was created and received US $20 million, mainly from Japan, in hopes of discovering the main cause of health problems due to 131I radiation. These funds were divided between Ukraine, Belarus, and Russia, the three main affected countries, for further investigation of health effects. As there was significant corruption in former Soviet countries, most of the foreign aid was given to Russia, and no positive outcome from this money has been demonstrated.
Commemoration
The Front Veranda (1986), a lithograph by Susan Dorothea White in the National Gallery of Australia, exemplifies worldwide awareness of the event. Heavy Water: A Film for Chernobyl was released by Seventh Art in 2006 to commemorate the disaster through poetry and first-hand accounts. The film secured the Cinequest Award as well as the Rhode Island "best score" award along with a screening at Tate Modern.
Chernobyl Way is an annual rally run on 26 April by the opposition in Belarus as a remembrance of the Chernobyl disaster.
Cultural impact
The Chernobyl accident attracted a great deal of interest. Because of the distrust that many people (both within and outside the USSR) had in the Soviet authorities, a great deal of debate about the situation at the site occurred in the first world during the early days of the event. Because of defective intelligence based on photographs taken from space, it was thought that unit number three had also suffered a dire accident.
Journalists mistrusted many professionals (such as the spokesman from the UK NRPB), and in turn encouraged the public to mistrust them.
In Italy, the Chernobyl accident was reflected in the outcome of the 1987 referendum. As a result of that referendum, Italy began phasing out its nuclear power plants in 1988, a decision that was effectively reversed in 2008. A referendum in 2011 reiterated Italians' strong objections to nuclear power, thus abrogating the government's decision of 2008.